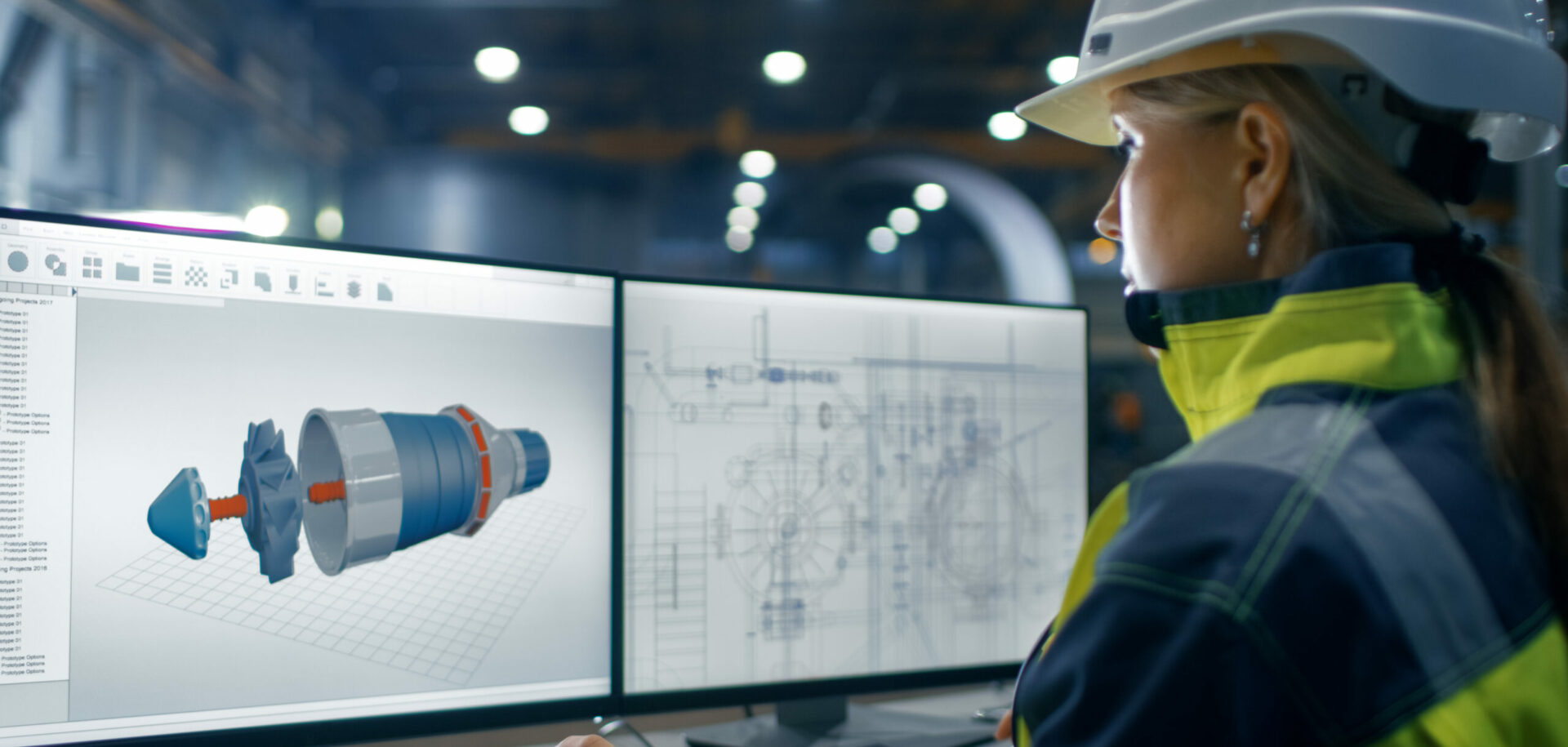
Grease lubrication mechanisms in rolling bearing systems
Grease lubrication has clear advantages over oil lubrication: Grease does not leak easily, it has sealing properties, and it protects bearing surfaces from corrosion. Its disadvantages are grease-life limitations and a limited cooling ability. Moreover, in some applications there is a risk of grease starvation, which leads to reduced lubricating films. However, if the right grease, sealing system and/or lubrication system are chosen, then grease lubrication offers clear benefits. This article, based on a new book written by SKF engineers [12], summarizes aspects of grease lubrication mechanisms in rolling bearings.
Grease lubrication offers many advantages compared with oil lubrication, but selecting the right grease is complicated. Understanding the lubrication mechanisms will help in selecting the right grease with the right amount for a specific application to meet bearing life expectations. To help understand grease lubrication, a new book written by SKF engineers was published in January 2013 by John Wiley & Sons Ltd [12] that covers the latest information and knowledge about grease lubrication. Grease lubrication is a dynamic process that can be divided roughly into three phases, as shown in fig. 1. After the initial filling or during relubrication, the grease will be located between the rolling elements, leading to high churning losses during start-up or running-in. During this phase, also called the churning phase, the grease will be pushed into the unswept volume of the bearing (onto the seals or onto the bearing ring shoulders) or will end up attached to the cage. From these locations, the grease will slowly provide the raceways with lubricant by either bleeding or shear. In this second phase, the bleeding phase, the lubricating film will be governed by a feed and loss mechanism [20] in which the raceways are fed by grease from the reservoirs but also lose lubricant due to side flow and oxidation. This may lead to starvation, especially in sealed bearings where the grease reservoirs are smaller. Another feed mechanism is occasional replenishment caused by softening of the grease close to the contacts due to local heat development [14], which is again caused by occasional film breakdown. At some point, the reservoirs may be empty or deteriorated to the point that replenishment can no longer happen. If relubrication has not taken place, severe film breakdown will result, called the end of grease life, which subsequently leads to bearing damage and failure. The rate at which the reservoir formation will take place is governed by the flow properties of the grease, also called its rheological properties. This will also determine the physical degradation of the grease. Lubricating grease shows visco-elastic behaviour, meaning that the viscosity of the grease is a function of both shear and shear rate. Fig. 2 shows viscosity as a function of shear rate in terms of the various models that are often used. It shows that the viscosity is very high at low shear rates. This means that the resistance to flow will be very high if the grease is not touched – i.e., when it is located in the upswept volume. This property is also called consistency. During the churning phase the grease may lose some of its consistency. This property is called mechanical stability. The viscosity of lubricating grease is so high at very low shear rates that only creep flow will occur, and the grease has an apparently solid behaviour. As shown in fig. 2, grease shows shear thinning, which is when the grease viscosity decreases substantially with increasing shear. At very high shear rates the grease viscosity may approach the base oil viscosity. Such high shear rates occur in the lubricating films between rolling elements and raceways. Together with oil bleeding this is the reason why the film thickness in grease-lubricated bearings is usually calculated using the base oil viscosity, ηoil. Both base oil and thickener are known to enter the bearing [2]. The lubricating film thickness in grease-lubricated bearings is determined by boundary layers formed by thickener material hR and by the hydrodynamic action of the base oil hEHL (elastohydrodynamic lubrication, EHL) [4]). For the latter, the base oil can be also taken into account for the reasons mentioned above. The film thickness, hT, is therefore: hT = hR + hEHL (1) Grease-lubricated bearings often run under so-called starved lubrication conditions where only very thin layers of oil are available and where the film thickness is primarily a function of the thickness of these layers (fig. 3). The change in thickness of these layers is given by the difference between the feed (bleeding [3, 21]) and loss flow rates of lubricant into or out of the raceways [20]. The oil in the track is lost due to the transverse flow caused by the high pressure inside the rolling element-raceway contacts [18]. Some replenishment may take place [6]. However, except for very low speeds and low-viscosity base oils, this will be a very slow process [7]. Shear and drag due to ball spin will probably have a greater effect [5]. Both ball spin and the narrower contact sizes make replenishment easier for ball bearings than for roller bearings. This is one of the reasons why roller bearings require grease with more bleeding than ball bearings [11]. At higher temperatures oxidation and evaporation will have an impact on the film thickness. Material will be lost due to oxidation and evaporation [19, 15]. However, this will also change the viscosity and lubricity. Starved lubrication will cause a decrease in film thickness that will proceed until the bearing is no longer well lubricated. Metal-to-metal contact will cause bearing damage or may cause enough heat development to occur to reduce the viscosity of the lubricant close to the contact for replenishment, leading to an event. In the latter case, the film thickness will increase again, resulting in sufficient lubrication until the next event takes place. This may occur a number of times, depending on the healing ability of the grease, which is a function of the ability of the grease to maintain its fluidity. Fig. 4 shows an example of the temperature profile of a cylindrical roller bearing running under self-induced temperature [14]. Grease life is defined by the point in time where the grease can no longer lubricate the bearing. This time may be very long and therefore difficult to measure in a bearing test rig. To accelerate such a test, the outer ring of the test bearing is heated, which accelerates the ageing process and reduces the viscosity of the grease. An example of such a test rig is the R0F+ grease life tester [13]. Lubricating greases are developed to operate in a limited temperature window. The maximum temperature, called high-temperature limit (HTL), is determined from the dropping point when the grease loses its structure irreversibly. This temperature may not be exceeded at any time. The safe maximum temperature is lower, called high temperature performance limit (HTPL). The low temperature limit (LTL) is determined by the temperature at which the grease will enable the bearing to start up without difficulty. It is usually measured by a start-up torque test. The safe minimum temperature therefore is higher, called low temperature performance limit (LTPL) [1]. In the zone between these safe temperatures the grease life is a function of temperature where, as a rule of thumb, grease life halves with every 15 °C temperature increase. Various models exist that can be used to predict grease life (or relubrication intervals). All models are empirical, based on grease life tests. Grease life is defined as the L10 life: the time at which 10 % of a large population of bearings have failed. Relubrication should be carried out before the end of grease life so that no damage to the bearing has taken place. The SKF relubrication model is based on L01, assuming L10 = 2.7 L01, which is the time where only 1 % failure would take place. Relubrication is not straightforward. Too much grease will keep the bearing in the churning phase, with high frictional losses and high temperatures. Fig. 5 shows the grease life for lightly loaded capped deep groove ball bearings as a function of rotational speed, mean bearing diameter, operating temperature and grease type (grease performance factor). Correction factors can be applied for the impact of load. Models for other bearing types are based on this model where other correction factors are applied. These can be found in the SKF rolling bearings catalogue [1]. Both the mechanical and chemical properties of the grease will change while the grease is exposed to milling and oxidation in the bearing. The type of oxidation depends on the operating conditions: Physical ageing dominates at lower temperatures and higher speeds, whereas chemical ageing dominates at high temperatures [9]. Physical ageing results in a change to the grease’s rheological properties, which results in leakage, reduced bleeding properties and a reduction in its ability to replenish the contacts. Chemical ageing is primarily caused by oxidation. Anti-oxidants retard this process, but when these are consumed, oxidation leads to a loss of lubricant due to a reaction into volatile products and to lacquer formation, which no longer lubricates the bearing [9]. The main differences between grease and oil for seal lubrication are related to starvation (replenishment of the seal lip) and boundary film formation by the thickener material. Replenishment of the contact is caused by shear and oil bleeding from the grease. The sealing action of grease is ascribed to the stiffness of the grease, which means that the grease will not easily flow away from the sealing contact. In addition, the grease will form pockets in multiple-lip seals where the flow of contaminant particles will be very slow. In the case of pressure differences across the seal, it will only be a fraction of the grease that will flow and cause migration of contaminant particles. In situations in which the grease cannot provide the bearing with sufficient service life or in the case of contamination by particles or water, relubrication may be applied by means of lubrication systems. These systems consist of pumps, pipes, valves, distributors and controllers. There can often be competing properties of grease as to what is good for a lubrication system to pump and what is good for the bearing. The lubrication system should be designed to handle a grease that is best suited for the bearing. The design is given by the flow properties of the grease, also called pumpability. SKF has developed a grease pumpability test programme that considers various aspects, which are listed below with the SKF test method in parentheses: 1. Delivery 2. Flow ability 3. Oil separation (and hardening): To measure the bearing (lubrication) condition online, generally vibration levels are measured. However, increasingly acoustic emission techniques [16] are used. Off-line techniques to measure the grease condition are oil bleeding, oil content, consistency, particle contamination and oxidation (FTIR spectroscopy). Methods are available to determine the remaining grease life with the results of these methods [10]. Over the past few decades SKF’s knowledge about grease has improved substantially. Today, it is possible to predict to a good extent the lubricating life of grease and to monitor its remaining life. Sealing prolongs the service life of the bearing in contaminated environments where the grease provides an additional sealing effect. Lubricating systems can be used to provide the bearing occasionally with fresh grease.
Summary
Phases in grease lubrication
Grease reservoir formation
The grease rheology can be described using various models depicted in fig. 2.Film thickness
Dynamic behaviour
Grease life and relubrication
Safe operation
Grease life models
Ageing
Grease lubrication mechanisms in seals
Lubrication systems
(a) Flow resistance (FTG5 and Lincoln Ventmeter)
(b) Compressibility (FTG1)
(c) Pressure Venting (FTG3 and Lincoln Ventmeter)
(a) Flow pressure
(b) Unworked penetration
(c) Deliver index for the pump unit (FTG4)
(d) Functioning of the pump unit
Grease hardening under pressure (FTG2).Condition monitoring
Conclusions