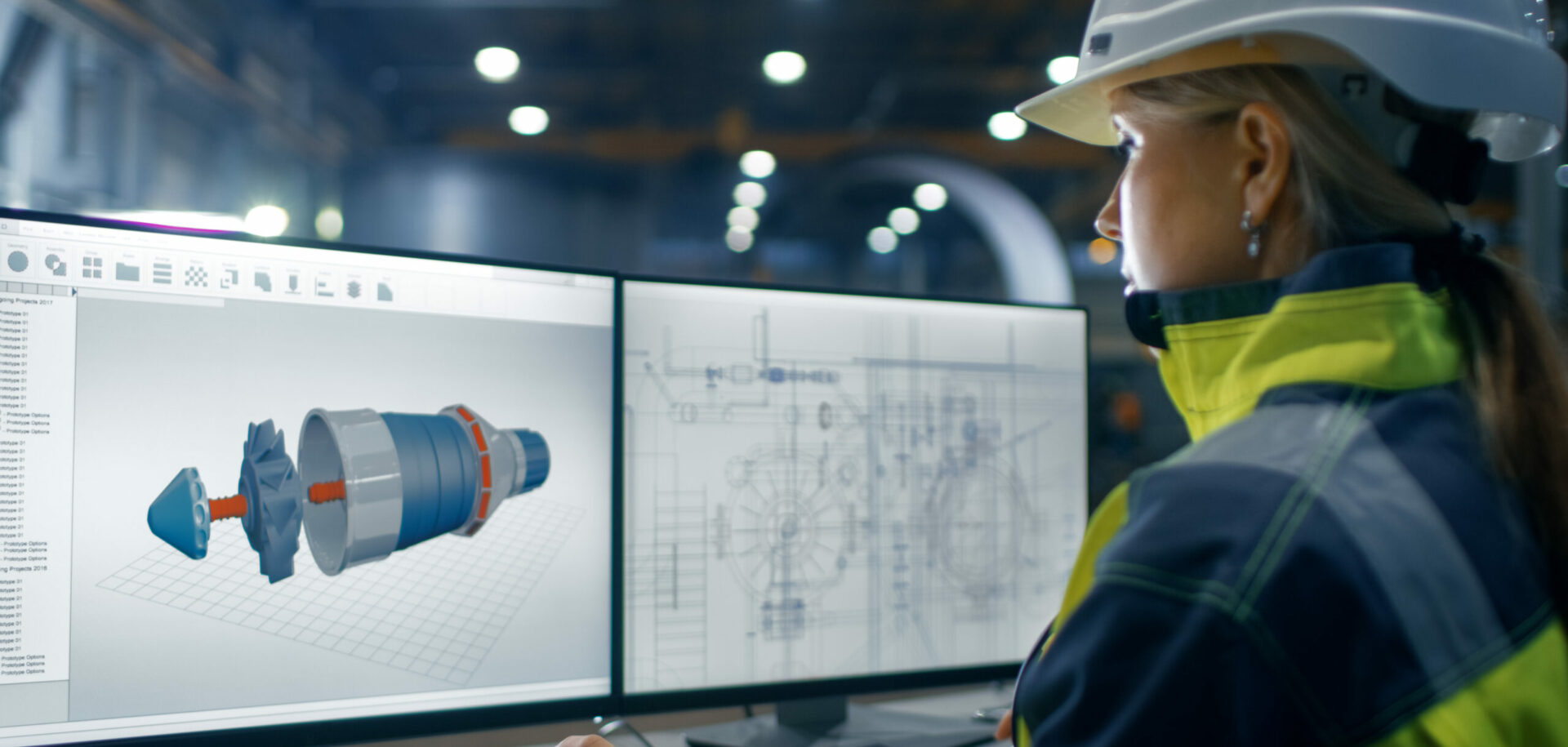
A heavenly view
Summary
Heavy-duty shoes
Telescopes need bearings that are stiff, precise and free of friction. The Gemini units also require bearings able to handle a weight of 342 metric tonnes rotating on the vertical (azimuth) axis and 172 metric tonnes on the horizontal (elevation) axis. Each Gemini telescope uses hydrostatic shoe bearings from SKF.
Hydrostatic shoe bearings are normally used for applications that handle very heavy loads, such as the drums that grind ore and cement and the debarking drums used in pulp production. “Because of their construction, these self-aligning bearings do not wear out”, says Alf
Sellin, senior application engineer for hydrostatic shoe bearings at SKF. Pressurised oil is pumped between the shoes on the support surface and the moving surface until a load-carrying film of oil is formed and the runner lifts off. Contact between the sliding surfaces is avoided, and there is virtually no friction at low or high rotational speeds.
Telescopes are sensitive to changes of temperature, explains Sellin. Because it is easy to regulate the temperature of oil, it works as a thermostat to help keep the temperature stable in both the telescope and its environment.
On each Gemini telescope, six azimuth hydrostatic shoe bearings (measuring 410 mm by 530 mm on the surface) are situated around the radial surface of the base ring, and the unit is held in place with six guiding pads. On the elevation axis, four bearings (with surface areas of 120 mm by 270 mm), along with two guiding pads, help the telescope tip freely.
The Gemini telescopes will see farther into space than ever before in the history of astronomy, even penetrating space clouds to watch stars being born.From the summit of the highest mountain in the Pacific Basin and in the foothills of the Chilean Andes, a pair of twin telescopes is making history.
Built by an international venture known as the Gemini Project, the 8.1-metre telescopes will allow astronomers to study the entire sky from pole to pole. “These telescopes will be able to do things no other telescopes have been able to do,” says Peter Michaud, public information and outreach manager of the venture. “We’ll be able to see more clearly than ever before.”
Gemini North, near the top of 4,200-metre-high Mauna Kea in Hawaii, and Gemini South at Cerro Pachón, Chile, are at two of the best astronomical sites in each hemisphere. When the units are fully operational, they will use new technologies that should allow them to spot features smaller than 0.07 of an arc second (an arc second is 1/3,600 of a degree) in the near infrared of deep space – better than the Hubble Space Telescope can manage at optical wavelengths with its 2.5-metre mirror.
Lightweight structures
The structure of each Gemini “light collector” is sparse and light compared to the bulky mass of the Palomar telescope that dominated astronomical research in the 1950s and 1960s. At the base of the Gemini is a concave 8.1-metre mirror just 20 centimetres thick. This mirror collects starlight, then reflects it up into a single, sharp dot on a small concave mirror about seven stories above. This secondary mirror, about a metre in diameter, reflects the beam of light back down through a hole in the centre of the large mirror. There, instruments collect and process the light and send it via fibre optic lines to computers at the base facility.
The light-gathering power surpasses that of past generations of telescopes because of the larger surface area of the primary mirror. The Gemini telescopes will be able to increase that power even more because of their extremely high image quality and infrared capabilities – features that make them unique in the class of 8 to 10-metre telescopes.
“Infrared is, pardon the pun, the hot topic for astronomy now,” Michaud says. Infrared imaging allows astronomers to see through the clouds of dust in space where stars and planets are formed.
It also allows increased ability to study expansion of the universe. As objects move away from us in space, their light appears to stretch out toward the red end of the spectrum. Through infrared viewing, scientists have seen galaxies speeding away. “It’s one of the main ways we know the universe is expanding,” says Michaud. “With the big aperture of an 8-metre mirror, we can look farther and see sharper and clearer. We hope it will give us more insight into the expansion and acceleration of the universe.”
Unique features
Much of the effort in building the Gemini telescopes has centred on the big mirrors. The glass was produced at Corning in New York and polished in France. Each piece was then transported carefully to its place in the observatory dome. Scientific American wrote, “No gemstone has ever been cut with greater symmetry. From peak to valley across its surface, the mirror varies typically no more than 16 nanometers (billionths of a metre). No point is more than 140 nanometers away from absolute smoothness.”
If each mirror were blown up in size to stretch across the Atlantic Ocean, and the slight surface variations were seen as waves, the tallest would be about 30 centimetres.
The mirror, weighing more than 22 metric tonnes, is supported partly by air pressure. Below it and around its edge, 180 hydraulic actuators actively control its shape. The actuators can make adjustments of the order of one-thousandth of the thickness of a human hair. The Gemini team also can coat the mirror with silver to decrease infrared radiation emissions from the mirror itself and improve reflectivity, thus improving infrared vision.
During operation, huge vents open in the 15-storey dome to allow night air to flow through, reducing thermal instabilities that distort starlight. The telescopes can also compensate for thermal turbulence that bends light and blurs images – the atmospheric turbulence that makes stars seem to twinkle.
One of the ways that Gemini increases the sharpness of images is by sampling the starlight up to 200 times per second and jiggling the secondary mirror to help keep the image stationary. The light is then processed through an adaptive optics system, which can further reduce the effects of turbulence and produce images that are near the theoretical resolution limit of the telescopes.
Scientific objectives
Funding for the USD 184 million project is coming from the United States, the United Kingdom, Canada, Chile, Australia, Argentina and Brazil. Because it is a public project, any astronomer from these countries can submit a proposal to use the Gemini telescopes. Among the topics the project hopes to explore are:
- Formation of planetary systems, of which current understanding is still rudimentary. Detailed mapping of the areas around young stars will allow astronomers to study the process of planet formation directly.
- Birth of stars. Stars are formed in dusty, obscured regions, which become more transparent at infrared wavelengths.
- Origin of quasars – amongst the most luminous sources known – as well as super-massive black holes, presumed to be at the centre of these sources.
- Formation and evolution of galaxies. The Gemini telescopes can gather spectral data from galaxies at greater distances than has been possible with the previous generation of telescopes.
- New directions for astrophysics. New discoveries can’t be predicted, of course, but as the Gemini Project notes: “Every time there has been a magnitude increase in observing capability, fundamental discoveries have been made which change the direction of astronomical research.”
A challenge to coordinate
One of the most challenging aspects of building the two Gemini telescopes has been the integration of diverse technologies. The idea, Michaud says, is “to make the telescope work like a living, breathing entity.” Optics, computers, electro-mechanical systems, hydraulic devices, sensors and monitoring devices, plus the software – the glue that holds it all together – must be coordinated to keep the telescope working for extended periods of time.
“All the systems must work together,” Michaud says. “If they don’t, then often nothing works.” Gemini North will be fully operational by the start of 2001. It made its first research runs this summer, while the telescope’s builders tweaked and adjusted it. It saw “first light” – the astronomer’s term for the first time a telescope focuses on the skies – in January 1999.
Building is going even faster at Gemini South. Because it’s an identical unit, most problems builders faced putting up Gemini North, such as software bugs or control devices, have already been solved, says Michaud. First light is expected by the end of this year, with full operations by the second half of 2001.
In June 1999, Gemini North, at the top of Mauna Kea, was dedicated. The promise of the Gemini Project, and the hope its crew has for the future, was well summed up by Rita Colwell, director of the US National Science Foundation. “We stand here on the brink of discovery that we cannot even imagine,” she said. “We can only be sure that these discoveries will enlarge our vision and make our spirits soar in this thin air and far beyond.”
Jack Jackson
An American freelance journalist based in Denmark
photos The Gemini Observatory