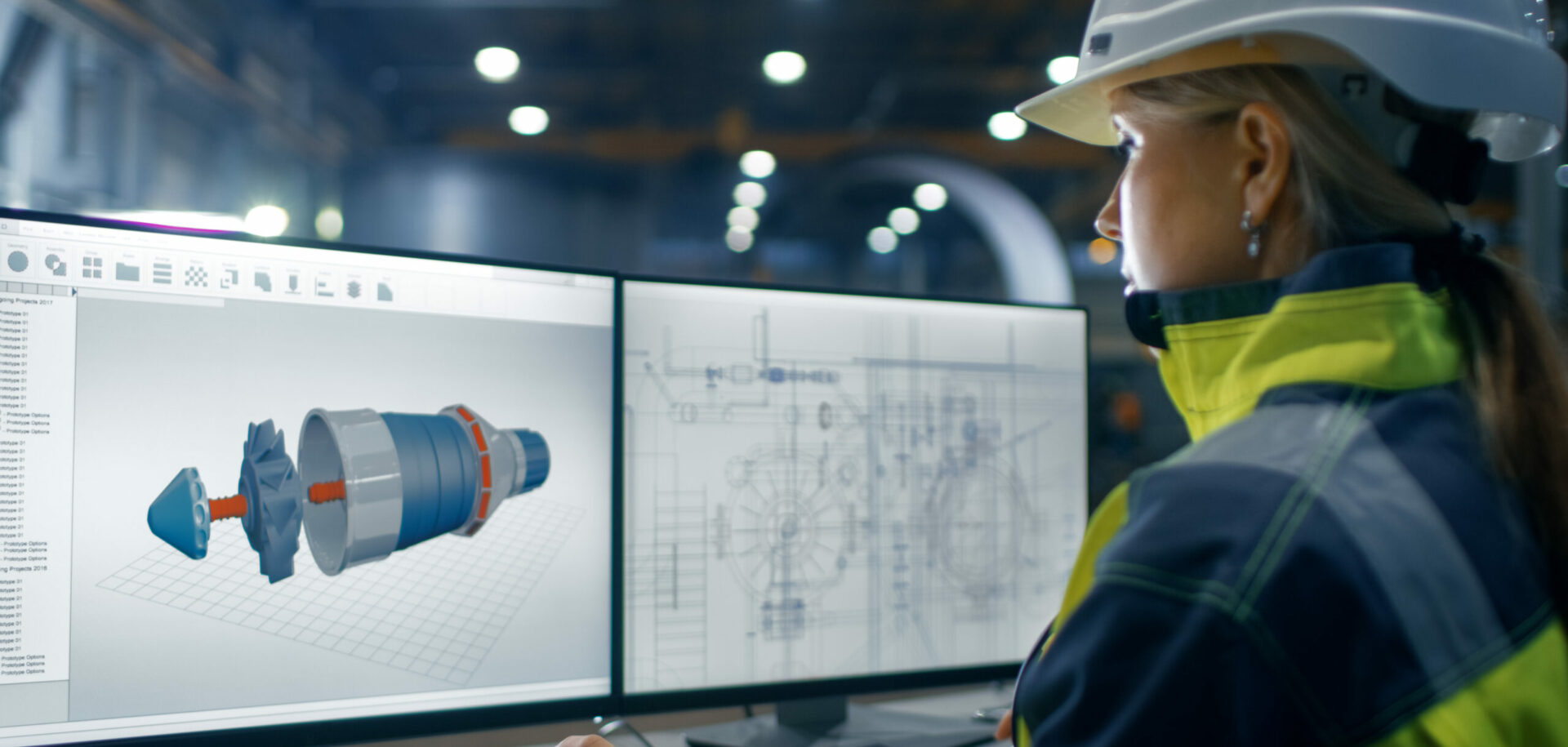
Noise and vibration in power steerings
Noise generated by car components represents a challenge to designers and manufacturers as they develop new generations of quiet vehicles.
Some types of noise can indicate irregular tribological conditions, which can lead to accelerated wear and component fatigue. Normally, noise is generated by a vibration of one or more of the components’ structural elements.
A friction-induced vibration is the result of instability during the friction of two rubbing parts. For this to occur, the friction force should depend on the relative speed of the two rubbing components and decrease with increase of this speed.
Focusing on noise in the pinion seal, researchers were seeking to develop a criterion to predict the occurrence of noise when rubber or plastic slides on a solid surface.
Investigating any noise-related problem is fraught with difficulties. One challenge is reproducing the noise in the laboratory environment. Another is that noise usually has many sources and is masked by structure-borne or structure-amplified vibrations. Apparently, in this case, it happened when noise from the steering pump and other mechanisms excited the same natural frequencies of the structure as that of seal noises.
The steering gear noise proved to be very elusive. It occurred only in a very small number of cars and was extremely unstable, appearing one day and disappearing the next. It had been established that steering oil temperature and oil pressure were among the factors affecting this phenomenon.
When noise was reproduced under laboratory conditions, it was also necessary to confirm that this noise matched the field condition noise. For this reason, the investigation was carried out in three phases. The first task was to record noise under field conditions, identify the noise signature and establish the noise source. The next step was to reproduce this noise in the laboratory and confirm its authenticity. Finally, the most important factors in noise generation were identified, noise mapped against these factors and measures for its elimination proposed.
Field observations
Steering gear noise was recorded under field conditions for different loading situations. The noise had a characteristic low-pitched “ziz” sound. It was established that the noise would normally begin once the car was completely warmed up. Also a steering pressure increase aggravated the noise. The noise was then analysed using SKF Condition Monitoring Microlog instruments. The frequency analysis of the observed noise is shown in fig. 1. The noise spectrum is generally a very useful tool for the analysis of harmonic oscillations, appearing on the graph as a stand-alone peak. Even if the noise is masked by other noises, the background noises can be cancelled out from the frequency spectrum by comparing cases with, and without, the noise. However, the “ziz” noise happened to be a non-harmonic vibration, which made frequency representation practically useless.
The character of the noise can be seen from time domain recordings. Fig. 2 reveals a number of compact groups of sound intensity peaks, spread over time. Each group represents the noise occurring during one turn of the steering wheel. The intervals between the groups indicate the time between the change in one direction of steering to another. Looking more closely at any of these groups reveals the nature of the sound.
Fig. 3 reveals the “ziz” sound to be the consequence of an almost periodic series of events, most characteristic of a stick-slip condition. The periodic set of square marks inserted with a period of 0.0507 seconds is almost coincident with the individual peaks. The estimated frequency of stick-slip events is 19.7 Hz. As each event is a sharp peak in the time domain, it becomes clear why the frequency domain was not convenient for analysis. Indeed, any peak-like effect in the time domain would decompose into a wide distribution of frequencies. Those corresponding to the structural resonance frequencies would be amplified, and the others, eventually, would be likely to be dampened. Consequently, noise spectrum analysis could only give information on resonance frequencies and other harmonic excitations.
Time domain representation provides an easily recognisable signature of this noise. If noise encountered in a simulation experiment displays the same, or similar, time domain behaviour, it can be assumed that this is the same noise as in a vehicle.
Noise source
To identify the noise source, the “noisy” steering gears were removed from the cars and analysed in a laboratory. A special “quiet” room was built to isolate them from environmental noise. The steering pump, as one of the significant noise contributors, was installed remotely from this room with the supply of steering fluid pressure carried out through flexible tubing. A test rig was built to adapt the steering gear (fig. 4). This rig allowed experiments with controlled steering cyclic speed, pressure, oil temperature, axial and side rack loads. Measurements, including the torque and the noise, were taken to identify the noise source. A few areas were suspected initially: pinion seal, input seal, inboard and outboard rack seals, piston seal. After initial tests, only two suspects remained – the rack seals and the pinion seal. A matrix experiment (Table 1) allowed the rack seals to be eliminated, leaving only the piston seal. Laboratory tests confirmed the noise signature to be similar to that in field tests.
Noise mapping
A pinion tower simulator was constructed for noise studies. The tested seals were either installed in a specially designed steel chamber, or in a pinion tower cutout. This cutout contained two seals – input and pinion. The simulation chamber had two pinion seals installed. A pinion shaft with an outside diameter equal to 23.125 mm was connected to a variable speed electric motor through a torque meter and a flexible coupling. In this way potential vibrations coming from the motor and the foundation are isolated.
Oil circulated between the chamber and a heater was installed to maintain the oil temperature. Shaft speeds were selected in the range from 1 to 30 r/min as the most representative for the power steering application. The oil pressure was controlled by a compressed nitrogen system using a set of valves and a pressure gauge. To model the actual steering gear conditions, the seals had a sprung radial load of 2.189 N/cm for HNBR (hydrogenated nitrile rubber) compounds and 2.736 N/cm for FKM (fluoro rubber) materials. The seals were installed with a tightly controlled shaft-to-bore misalignment of 0.125 mm and a dynamic run-out of 0.125mm.
Noise was detected audibly using microphones and via a torque meter. Initially the noise proved very difficult to reproduce until a special installation procedure was developed. Under normal circumstances, even the noisy seals from the field would not show any vibration in the simulator. Only when seals were chemically cleaned before the installation did the noise become reproducible. Noise was almost always present when the oil temperature was 71 ºC (160 ºF) and the pressure was 0.54 MPa (80 psi) – the normal application temperature and pressure.
The signature of the noise from the simulator was also checked against the characteristic signature of the cause and was found to relate. The torque meter chart recordings when the shaft speed was progressively increased is shown in fig. 6. There are two sets of lines on these charts. The longer background vertical lines register the teeth of the gear on the shaft when they pass a proximity sensor. This arrangement was used to ensure accurate measurement of the shaft speed and identify each phase of rotation at which the noise began and ceased. The second line was for the torque measurements. This was so dense that it appeared as a band on the chart. The position of this band relative to the zero-level indicated the torque level.
The torque changes in time almost periodically because the lip radial load changes due to the dynamics run-out installation condition. The noise could be determined both by widening of this band and by audible means. Under normal application conditions, noise begins between approximately 4 and 6 r/min. At 11 r/min, the noise became erratic and at 15 r/min, it stopped. The next set of tests was performed on a pinion tower simulator with seals manufactured from the two different materials – FKM and HNBR-based rubber compounds. The seals were tested at a range of temperatures. Noise maps are shown in figs. 7 and 8.
Discussion
Noise is difficult to reproduce. Indeed, in the actual pinion tower, the noise began at 6 r/min and stopped at 15 r/min, while in the simulator, it was registered almost entirely throughout the whole test range of 5 to 30 r/min. In other cases, the same simulator test, using a different set of seals, could produce slightly different results than those shown in figs. 7 and 8. Analysis of the shaft torque (fig. 9) provides an explanation. In a range of shaft speeds from 4 to 15 r/min, the torque decreases as the speed increases. This is a necessary condition for the instability of a harmonic oscillator with friction, leading to a periodic excitation perceived as a noise. A seal lip is not exactly a harmonic oscillator but it can be mathematically modelled as such. At 15 r/min, the torque derivative becomes positive and the condition for noise is violated, so the noise stops.
The fact that noise did not occur in one set of experiments and did in another is not surprising. It is well known that the friction force is a poorly reproducible property. The shaft speed range with a negative torque derivative can vary from seal to seal. Besides, the negative derivative of the torque curve is only the necessary, and not the sufficient, condition for the instability. Some research indicates that under certain conditions, the initial perturbation can be suppressed by the friction, even if the instability condition is satisfied. By providing random alterations to the contact surfaces, impurities can also cause conditions that result in noise suppression. This can explain why noise was difficult to reproduce for non-cleaned seals.
The negative derivative of the torque as an instability condition can not predict the noise occurrence. When the derivative is positive, however, noise cannot occur. This can be illustrated by testing HNBR seals of exactly the same geometry as the FKM seals. Under typical applications where temperature was 82 ºC (180 ºF) and pressure 0.13 – 0.54 MPa, the HNBR seal had a positive derivative so noise was impossible. This criterion proved helpful in identifying, and so preventing, situations susceptible to noise. The criterion arose from the analysis of a simple oscillator with friction depending on the friction speed. A theory behind the formulation of the instability criterion for a rubber has been developed by the author but is not discussed in this article. This theory was presented at the Society of Tribologists – Lubrication Engineers 53rd Annual Meeting.
Key to this theory is the description of this criterion in the form of the following inequality:
where is the stress phase shift angle for harmonic distortions – a rubber material characteristic, ln (VS) is a natural logarithm of vs – the surface speed of the shaft, and F (VS) is a frictional force, being a function of the surface speed VS.
Meeting the condition indicates instability, and, therefore, susceptibility for noise. The violation of the inequality marks a quiet regime.
The friction coefficient computed for the pinion seals varies significantly but is almost always more than 0.5. This indicates that the seal lubrication condition achieved in the application is essentially dry or “sparse” boundary lubrication. For all rubbers, friction changes with speed and exhibits a peak-like behaviour. The position of the peak is an attribute of the rubber and depends on temperature and the texture of the frictional surfaces. In studies, it has been found that the position of the peak is related to the glass transition temperature of the rubber compound. At the speed range above the friction peak speed, the friction derivative is negative and, therefore, the rubber part becomes susceptible for noise. To avoid noise occurrence, a rubber compound has to be selected with a friction-peak speed well above the application parameters’ range. The HNBR rubber compound, used in the tests, displays such a property and, consequently, is a better material for this application than the FKM compound.
The HNBR (fig. 10) material has other benefits when compared to FKM. As the FKM compound has less affinity toward the lubricant than HNBR, then retention of the oil film is superior with the HNBR compound. For as long as the lubrication condition is not dry or sparsely boundary lubricated, noise will not be possible. This adds to the attractiveness of HNBR compounds for steering pinion applications.
Conclusions
Pinion seals are the cause of the “ziz” noise. Pinion seal noise was reproduced under laboratory conditions, with extensive noise mapping carried out. This noise occurred because of lack of lubrication under the lip and due to the unfortunate match between the range of parameters, shaft speed, oil temperature and pressure, where FKM rubber compounds generate noise and the range of parameters typical for the steering application.
Based on noise analysis, it has been recommended to switch the rubber in favour of a carefully selected HNBR material. Finally, a new criterion for vibrational instability was derived and verified. This works over a large range of operational parameters.
Alexander Berdichevsky
Chicago Rawhide, Chicago, Illinois, USA